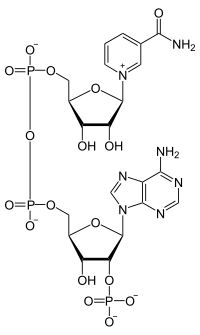



NAD, NADP or NAD(P)? The presence of the additional phosphate in NADP presents a significant challenge for an NAD-specific enzyme: such a lot of additional negative charge! In the opposite sense, an NADP-specific enzyme might require a strong set of interactions with the phosphate moiety in order for the enzyme to grasp the substrate (or indeed the transition state), during hydride transfer. In general, enzymes are specific for one or the other cofactor, whilst some like GDH in some species, can handle both with similar effieciency. There is a general rule of thumb however, and that is that anabolic dehydrogenases tend to be NADP-specific, whilst catabolic dehydrogenases prefer NAD. As always, rules of thumb in Biology can be your undoing!
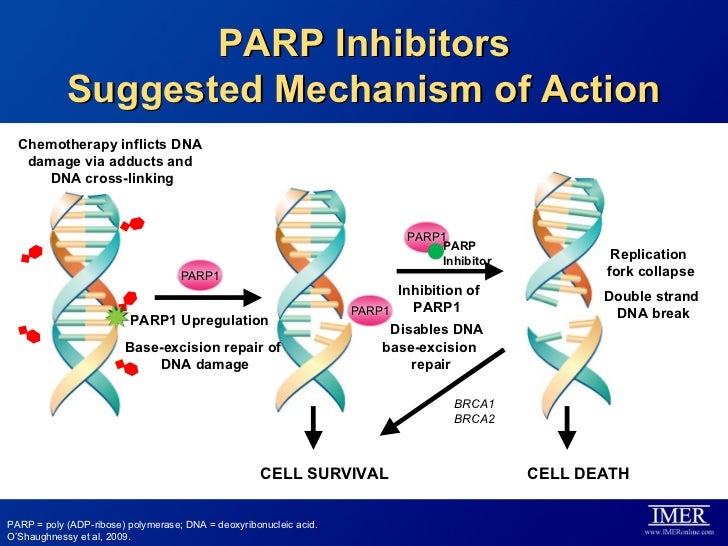

I hope you agree with me that NAD as a suitable candidate as a molecule of the month!